The McKnight Endowment Fund for Neuroscience has selected four projects to receive the 2023 Neurobiology of Brain Disorders Awards. The awards will total $1.2 million for research on the biology of brain diseases, with each project receiving $100,000 per year in each of the next three years for a total of $300,000 funded per project.
The Neurobiology of Brain Disorders (NBD) Awards support innovative research by U.S. scientists who are studying the biological mechanisms of neurological and psychiatric disorders. The awards encourage collaborative and cross-disciplinary applications that could potentially lead to laboratory discoveries about the brain and nervous system and thus diagnoses and therapies to improve human health.
An additional area of interest is the contribution of the environment to brain disorders. Early-life environmental stress is a powerful disposing factor for later neurological and psychiatric disorders. Studies show communities of color are at higher risk for these stressors, which range from environmental (e.g. climate, nutrition, exposure to chemicals, pollution) to social (e.g. family, education, housing, poverty). From a clinical perspective, understanding how environmental factors contribute to brain disease is essential for developing effective therapies.
“This year’s group of McKnight Neurobiology of Brain Disorders awardees are at the forefront of brain research and have already made some stunning discoveries,” said Ming Guo, M.D., Ph.D., chair of the awards committee and Professor in Neurology & Pharmacology at UCLA David Geffen School of Medicine. “The work they are doing has the potential to lead to treatments for ALS, epilepsy, obesity, and brain cancer—devastating illnesses that affect millions of people worldwide.”
The awards are inspired by the interests of William L. McKnight, who founded The McKnight Foundation in 1953 and wanted to support research on brain disease. His daughter, Virginia McKnight Binger, and The McKnight Foundation board established the McKnight neuroscience program in his honor in 1977.
Multiple awards are given each year. This year’s four awardees are:
Assistant Professor of Neuroscience, Yale University School of Medicine, New Haven, CT
Mechanism and functions of repeat expansion self-exonization in C9orf72 ALS/FTD
Assistant Professor of Neurology, Stanford University School of Medicine, Palo Alto, CA
Neuron-to-OPC synapses in adaptive and maladaptive myelination
Associate Professor, Basic Sciences Division, Fred Hutchinson Cancer Center, Seattle, WA
Adipocyte-brain mitochondrial signaling and its impacts on brain function
Assistant Professor of Neurology, Brigham and Women’s Hospital & Harvard Medical School, Boston, MA
The neurobiology of glioma: Understanding malignant neural circuits instructing tumor growth
With 164 letters of intent received this year, the awards are highly competitive. A committee of distinguished scientists reviews the letters and invites a select few researchers to submit full proposals. In addition to Dr. Guo, the committee includes Sue Ackerman, Ph.D., University of California, San Diego; Susanne Ahmari, M.D., Ph.D., University of Pittsburgh School of Medicine; Andre´ Fenton, Ph.D., New York University; Tom Lloyd, M.D., Ph.D., Johns Hopkins Medical School; and Harry Orr, Ph.D., University of MN.
The deadline for Letters of Intent for the 2024 awards is November 1, 2023.
About The McKnight Endowment Fund for Neuroscience
The McKnight Endowment Fund for Neuroscience is an independent organization funded solely by The McKnight Foundation of Minneapolis, Minnesota, and led by a board of prominent neuroscientists from around the country. The McKnight Foundation has supported neuroscience research since 1977. The Foundation established the Endowment Fund in 1986 to carry out one of the intentions of founder William L. McKnight (1887–1978), one of the early leaders of the 3M Company.
In addition to the Neurobiology of Brain Disorders Awards, the Endowment Fund also provides annual award funding through the McKnight Scholar Awards, supporting neuroscientists in the early stages of their research careers.
Neurobiology of Brain Disorders Awards
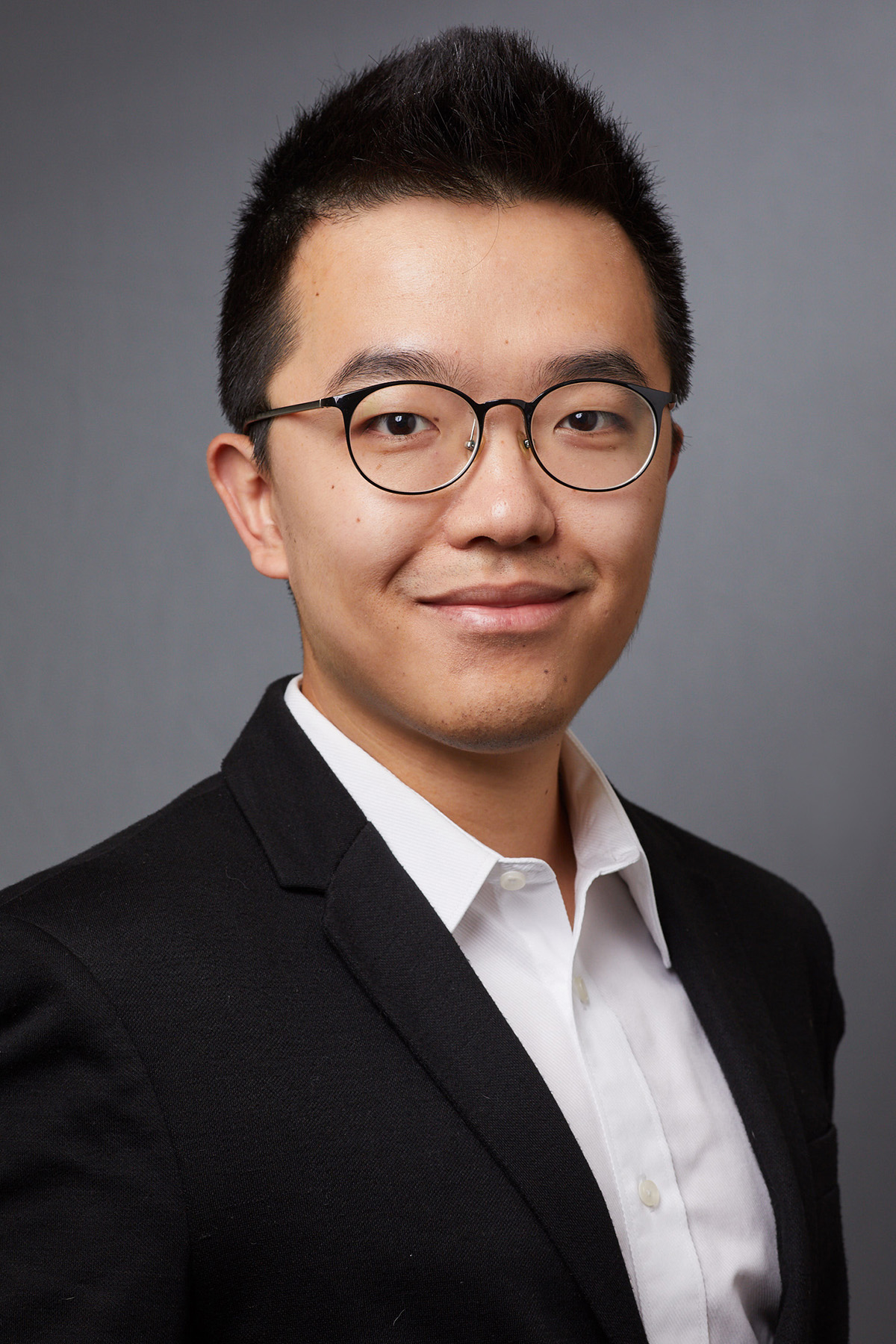
Junjie Guo, Ph.D., Assistant Professor of Neuroscience, Yale University School of Medicine, New Haven, CT
Mechanism and functions of repeat expansion self-exonization in C9orf72 ALS/FTD
As intricate as the DNA replication process is, sometimes errors happen. Some neurological diseases are linked to a particular type of error called nucleotide repeat expansion (NRE), in which a short DNA segment is repeated over and over in hundreds or more copies. Where these repeats occur in the genome matters: during a critical step in gene expression called RNA splicing, only certain pieces (exons) of the RNA transcribed from DNA are joined together to become the final messenger RNA, whereas the remaining RNA sequences (introns) between exons will be broken down.
However, in some cases, introns with NREs are not broken down, but manage to instruct the making of a variety of repeat proteins that are harmful to nerve cells. A well-known example is an intron NRE within a gene called C9orf72, which is the most common genetic cause of amyotrophic lateral sclerosis (ALS, or Lou Gehrig’s disease) and frontotemporal dementia (FTD). In his research, Dr. Guo hopes to uncover how this intron NRE disrupts RNA splicing and causes the production of toxic repeat proteins.
Guo and his team will first test a variety of NRE mutations to see which are able to change the splicing pattern so the intron can escape degradation. Their second aim will test the hypothesis that these changes in the splicing pattern are critical for the C9orf72 NRE RNA to increase its export out from the cell nucleus into the cytoplasm and instruct the making of toxic repeat proteins. Finally, their research will explore the possibility that differences between the ways in which each cell splices its RNAs may explain why certain types of nerve cells such as motor neurons are more vulnerable in ALS.
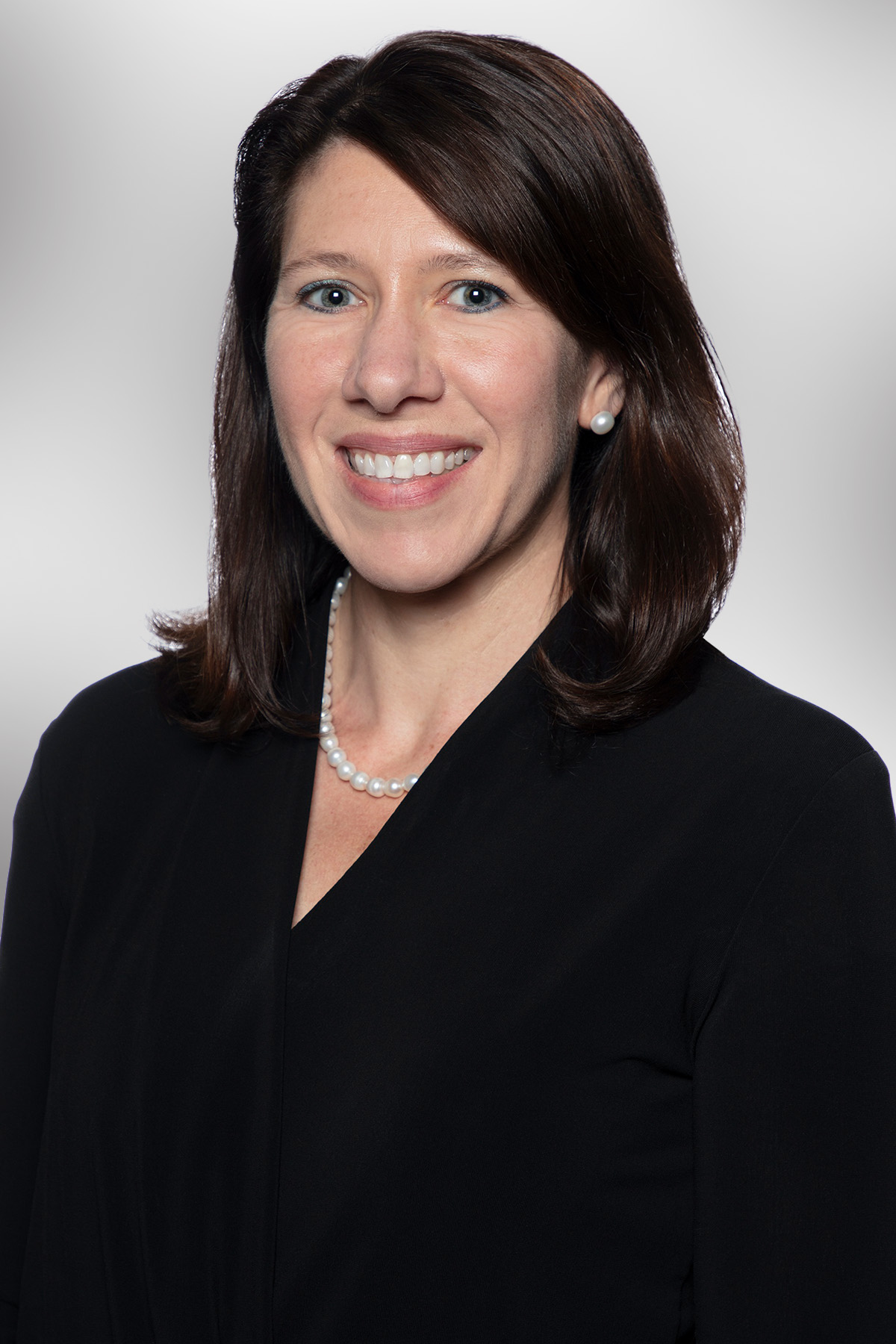
Juliet K. Knowles, MD, PhD, Assistant Professor of Neurology, Stanford University School of Medicine, Palo Alto, CA
Neuron-to-OPC synapses in adaptive and maladaptive myelination
In her role as a pediatric clinician specializing in epilepsy, Dr. Knowles sees firsthand how this neurological disorder (actually a collection of several related but distinct diseases) is experienced and how it progresses. As a neuroscientist, she has the opportunity to help uncover how and why. Knowles and her team are focusing their research on the role of neuronal activity in myelination in patients with generalized epilepsy, a common form of the disease that is characterized by the presence of seizures and absence seizures.
Myelination is the process by which the axons (projections) of neurons are encased in myelin, which enhances the speed of axon signal transmission, and makes neural networks more efficient. The process involves oligodendrocyte progenitor cells (OPCs) which can develop into oligodendrocytes, cells that produce myelin. In earlier research, Knowles uncovered that the neural activity of absence seizures promotes myelination of the seizure circuit, making it more efficient. This appears to lead to an increase in absence seizure frequency and severity; when Knowles and her team blocked the OPCs’ response to neural activity, seizure-induced myelination did not occur, and the seizures didn’t progress.
Knowles’ new research will now explore how this happens and identify possible approaches for future therapies. One aim will document the neuron to OPC synapses in both epileptic and healthy mouse models. A second aim will compare neuron-to-OPC synaptic activity and synaptic gene expression in healthy or epileptic mice – specifically focusing on how myelination promoted by a seizure differs from that promoted by learning. A third aim will explore how disrupting the post-synaptic receptors on oligodendrocytes affects the progression of epilepsy, not just in terms of seizures, but related symptoms such as disrupted sleep and cognitive impairment, both of which are common in individuals affected by epilepsy.
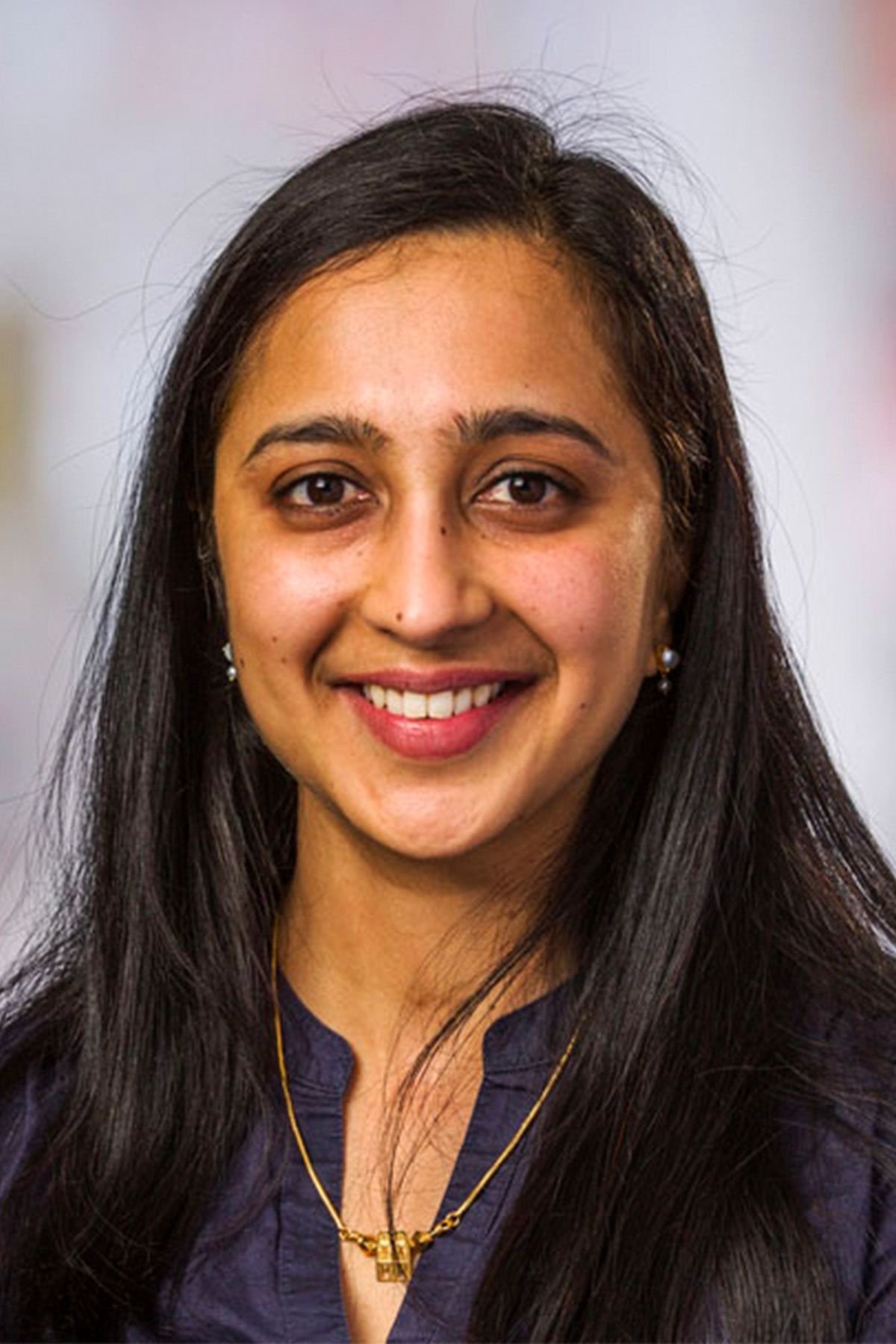
Akhila Rajan, Ph.D., Associate Professor, Basic Sciences Division, Fred Hutchinson Cancer Center, Seattle, WA
Adipocyte-brain mitochondrial signaling and its impacts on brain function
Communication between organs and the brain is critical to an animal’s survival and health. Signals tell the brain when the body needs more energy, is hungry, or needs to sleep, move or perform countless other tasks. But recent research has revealed that communication can include more than hormones – packets of material can also be passed to brain cells. Dr. Rajan’s research focuses on the phenomena of fat cells (adipocytes) sending bits of mitochondria – the organelles within cells that generate energy, among other roles – to the brain, and how that affects brain function.
Previous research has found that when these mitochondrial bits reach the brain, it makes the fly model Rajan’s team works with more hungry, specifically for high sugar foods, promoting a cycle of obesity and further sending of material. There is a known correlation between obesity and a range of neurological disorders, including sleep disorders and cognitive decline, and this new research hopes to shed light on these links and potentially identify targets for future therapies.
Working with the fly model, Rajan and her team aim to identify how exactly these bits of mitochondria are gaining access to neurons in the brain without being degraded; what happens when these bits of fat cell mitochondria integrate with neuronal mitochondria, specifically how it alters an animal’s behavior in terms of sleep and feeding; and what effect this process has on neuronal health overall. The research will take advantage of very precise genetic manipulations at which Rajan’s lab excels, involve cross-disciplinary insights provided by lab team members, and use advanced insect physiology chambers that let the team document feeding and changes in behavior at a level unavailable to previous generations of researchers.
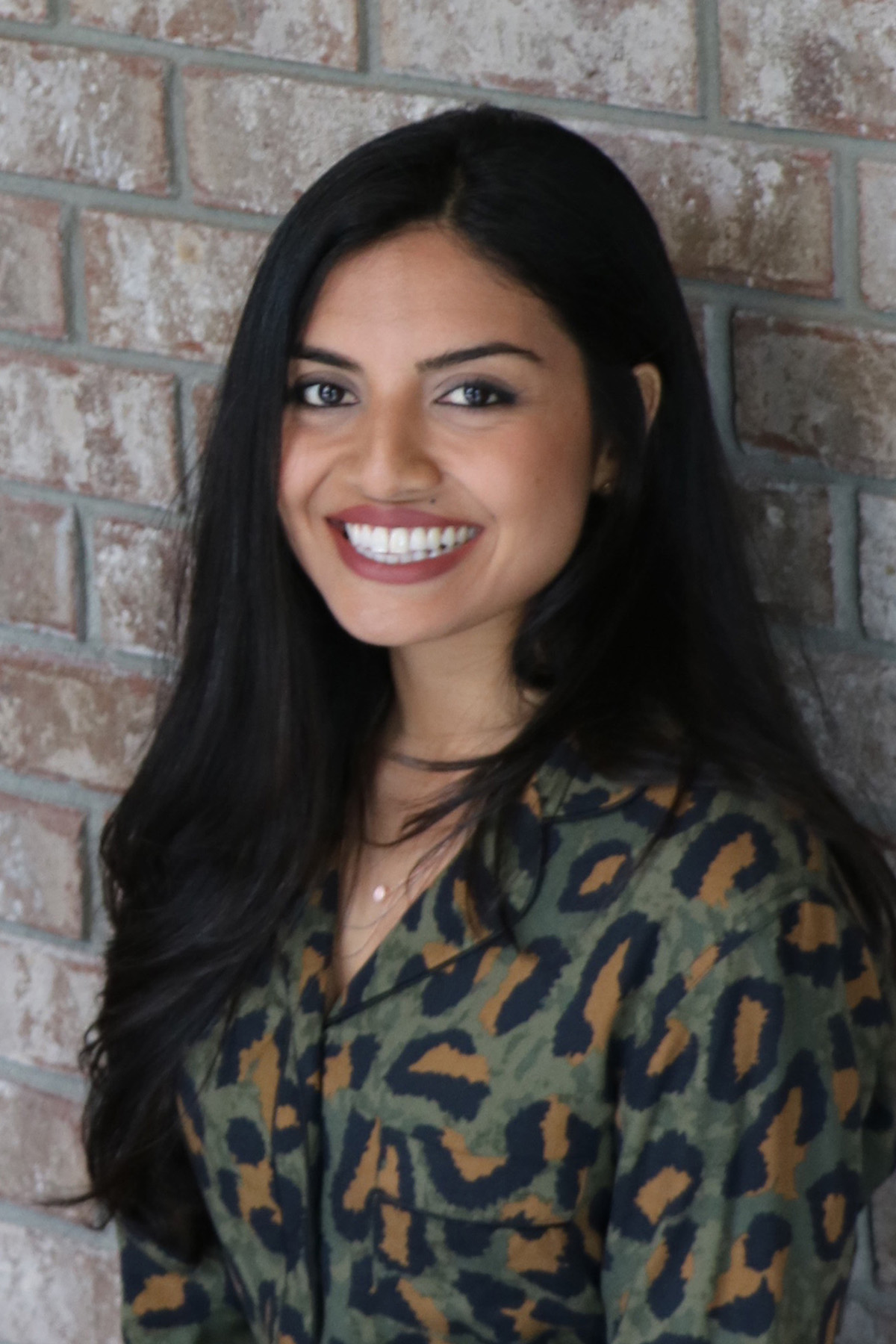
Humsa Venkatesh, Ph.D., Assistant Professor of Neurology, Brigham and Women’s Hospital & Harvard Medical School, Boston, MA
The neurobiology of glioma: Understanding malignant neural circuits instructing tumor growth
Cancers, including brain tumors, have traditionally been studied at a cellular or molecular level. Researchers are addressing questions such as what subpopulation of cells are involved, how do they mutate, and what can we do to those malignant cells to get them to stop replicating? Dr. Venkatesh is interested in looking at how the nervous system is also involved in cancer progression and has already discovered that neurons form synaptic connections to cancer cells.
Venkatesh and her lab are studying both primary and secondary brain tumors but have evidence that these findings apply to cancers in other parts of the body. The insight that tumors are interacting with neurons, and not just killing off nerves as had once been thought, has opened many possibilities. These malignant growths are taking signals from the nervous system intended to pass information to other cells and instead reinterpreting them to instruct the cancer to grow. Now researchers can explore how to harness the nervous system to help treat or manage this malignant disease. In an exciting development, Venkatesh’s previous work in this space has already led to clinical trials that repurpose existing drugs targeting the nervous system and apply them to cancer treatment.
This new research goes even further into understanding the mechanisms governing neural circuit activity-driven glioma progression. Using advanced neuroscience technologies and patient-derived cell lines, Venkatesh will be able to modulate and study the malignant neural networks, encompassing both neurons and tumor cells, that influence cancer growth. Understanding this activity-dependent mechanism and how it can be targeted without disrupting healthy neuronal function could open new fields of cancer research and novel therapeutic opportunities.